I’m an Emeritus Professor of Biology at the University of Washington, Seattle. Although I’m getting ‘long in the tooth,’ I still enjoying doing biological research and learning new approaches. Below are my main contributions – admittedly from my own viewpoint.
MY MAIN CONTRIBUTIONS
Thermal ecology
I’ve long been interested in finding ways to quantify and understand how body temperature influences the ecology and evolution of ectotherms. I first learned to appreciate the importance of temperature while spending 10-1/2 months studying the ecology lizards in the Kalahari Desert of southern Africa (between my MA and PhD, 1969-70). Previously, I’d done my MA thesis on interspecific competition and niche dimension complementarity in geckos in coastal Peru (only ~ 6°S), which was climaticaly buffered. But in the Kalahari, which was well into the temperate zone, I soon saw that daily and seasonal temperature variation – not interspecific competition – drove when and where lizards were active (Huey et al., 1977). That realization prompted me to switch from traditional ecology to one emphasizing thermal ecology.
My Ph.D. work was focused on the ecology of thermoregulation. Extending classic work by Rodolfo Ruibal and Stan Rand, I showed that forest species (and forest populations) of Anolis lizards were thermoconfomers, whereas open habitat species were thermoregulators (Huey 1974). Initially I found thermoconformity to be puzzling because 1) I’d seen Kalahari lizards thermoregulating very carefully and 2) the standard dogma of physiological ecology held that most lizards did and should thermoregulate carefully. These contrasting field observations (thermoregulation in Africa vs. thermocoformity or thermoregulation or even both in the Caribbean) inspired a theoretical model that incorporated the costs – not merely the benefits of thermoregulation (Huey and Slatkin, 1976). That model – plus background field studies – next inspired my attempts to measure “thermal performance curves”, which have since become a standard tool for evaluating thermal ecology and evolution in nature (see also Sinclair et al., 2016)).
I decided that sprint speed might be an ecologically relevant performance trait for lizards and thus suitable for measurements of thermal sensitivity. But how could I build an electronic drag strip? [Note: digital cameras did not exist then, and standard film would have been prohibitively expensive.] Fortunately, I met Walter Schneider, who was a fellow Miller and an electronics whiz. Walter helped me design and build an electronic drag strip (with TTL logic). Now I could measure acceleration and maximal speed as functions of body temperature! [Rob Stevenson and later Barry Sinervo hooked the drag strip to a computer. Many colleagues and I used this apparatus for many projects.] However, I must confess that my first ‘TPC’ was a plot distance jumped by a green frog as a function of temperature. This one-day project became a small chapter in my Ph.D. thesis at Harvard (1975), and was eventually published (see fig. 3 in Huey and Stevenson 1979). A botany grad student watched me jumping this frog in the basement of the Biolabs at Harvard. He commented: “Looks like a science-fair project.” True, but I got a lot of mileage out of that project.
[Note: During the same period, Al Bennett had independently built a drag strip and was measuring the thermal dependence of locomotor performance (speed and stamina) of lizards. We soon became close friends and great collaborators!] Over the decades, I’ve studied many aspects of thermal biology, including the consequences of retreat site selection, over-winter ecology, seasonal acclimation methodologies, evolution of viviparity, Jensen’s inequality and thermal preferences, ’hotter is better,” – see also AngillettaEA.pdf ), the first quantitative test of Janzen’s hypothesis, the first review of Janzen’s Hypothesis, and the 50th aniversary of Janzen’s hypothesis, evaluating ‘beneficial acclimation,’ – see also Huey et al. 1999, quantifying the extent of thermoregulation, and thermal dependence of demographic fitness.
I’ve enjoyed these studies immensely. In recent decades thermal biology has become a hot area of physiological ecology, evolution, and conservation. Unfortunately this came about as a consequence of climate climate change.
I had the good fortune to hear Dan Janzen lecture on why mountain passes were higher in the tropics. This was in Costa Rica in early 1966 during the first week of an OTS course on tropical ecology. Dan’s lecture was my first introduction to conceptual ecology, as I’d never had a real ecology course as an undergrad in Zoology. Dan’s talk made a deep impression on me, although I cetainly did not realize this at that time.
Years later, when I was finishing my Ph.D. thesis at Harvard (spring 1975), I happened to read a paper by Ron Heyer (1967), who noted that the elevational ranges of reptiles on a tropical mountain were narrower than those on a temperate zone transect in Yosemite. I immediately realized that this pattern was a logical expectation of Dan’s hypothesis. I spent an intense week in the library of the Museum of Comparative Zoology searching for and compiling distributional data of herps along multiple altitudinal transects. I found that indeed altitudinal ranges were narrower in the tropics, such that faunal overlap between elevations was greatly reduced. I included this analysis as a chapter in my Ph.D. dissertation (1975). The published version of this chapter was probably the first quantitative test of Janzen’s hypothesis.
In subsequent years I’ve been involved in two reviews of Janzen’s hypothesis: Cameron Ghalambor and colleagues Janzen’s Hypothesis and with Kimberly Sheldon and colleagues honoring the 50th aniversary of Janzen’s hypothesis. Dan’s paper has had a long-standing impact on our understanding of tropical and temperate-zone ecology.
Dan’s paper also got me thinking about what body temperature means to ectotherms, and that no doubt was a contributing factor to my inspiration to construct thermal performance curves and use them in ecological and evolutionary analyses. It even influenced my thinking about how oxygen levels over the Phanerozoic might have determined maximum altitudes of ectotherms over geological time as well as how high hypothetical “paleomountaineers” could have climbed over time (see below). In other words, mountains passes were higher in in the Triassic! In many ways, my career has been ‘variations on a theme by Dan Janzen.’
I was an early advocate of using phylogenies to design and analyze comparative physiological studies. Whereas most prior work contrasted physiological traits of distant relatives, I advocated working within clades and obtaining multi-species comparative data (see Huey and Pianka, 1981) and Huey 1987, in New Directions in Ecological Physiology.
Huey and Bennett, 1987 examined the co-evolution of thermal performance curves and thermal preferences in Australian skinks. This was the first attempt to look at comparative thermal physiology and coadaptation (behavior with physiology) in a phylogenetic context. We used a minimum-evolution algorithm (suggested by J. Felsenstein) to estimate temperatures at ancestral notes: thus we showed that the low thermal preference of some skink species was a evolutionarily derived trait.Garland et al., 1991. updated this analysis with a better phylogeny and using Felsenstein’s Independent Contrasts.
Peter Kareiva, Joel Kingsolver and I organized a large NSF-supported workhop on global and climate change. The resulting book (1993: ISBN 9780878934294) was among the first to call attention to global climate change. Subsequently, I’ve published many papers on related themes, especially as regards vulnerability of tropical ecotherms. These include: Deutsch et al., 2008, Huey et al., 2009, Dillon et al. 2010, Huey et al. 2012, Sunday et al., 2014, Deutsch et al. 2015, Buckley and Huey 2016, Sinclair et al. 2016, Deutsch et al. 2018, Huey and Kingsolver 2019, Huey and Buckley 2022.
A classical idea in evolutionary biology – championed by Ernst Mayr and others – holds that behavioral shifts drive evolution of other traits (“behavioral drive”). However, behavior can constrain rates of evolution. For example, some animals can use thermoregulatory behavior to evade or buffer environmental changes and associated physiological stress (Charles Bogert 1949, Evolution). Huey, Hertz, and Sinervo, 2003 developed a null model and showed how behavioral inertia (which we called the “Bogert Effect”) can potentially constrain or buffer evolution. But we also argued (see p. 363 in Huey et al., 2003) that even though thermoregulation can constrain evolution of thermal sensitivity, it can simultaneously drive the evolution of other traits (e.g., morphological ones). [Note: two decades earlier, Richard Lewontin (1983, Scientia 118:63-82) argued that homeostasis in one trait can be achieved only by “nonhomeostasis” in another trait. Our paper was thus a special case of Lewontin’s general point but added the concept of “behavioral inertia” on evolutionary rates.] Our paper won the President’s Award as the best paper published in The American Naturalist in 2003.
Martha Muñoz subsequently developed these themes as well as highlighted and greatly extended the general relevance of the Bogert Effect in evolution.
Around 1989 I became interested in experimental evolution of thermal sensitivity. I decided to work on Drosophila. At exactly the same time, Al Bennett - a close friend and colleague - independently decided to work with Rich Lenski on experimental evolution with E. coli. That we simultaneously switched from reptiles to flies or bacteria was rather disturbing to our herp colleagues, but our switches were not a coincidence. In large part through our collaborative studies together, we both recognized that comparative studies had limited relevance to predicting whether and how the thermal physiology of organisms would respond to contemporary climate change. We were both impressed by the power of experimental evolution, and we both felt that replicated laboratory experiments were needed to clarify genetic constraints on rapid evolution.
Left panel: In 1976 a friend sent this tea bag, which mimics a fortune cookie. To me, this one supports experimental studies of evolution. Right panel: Barry Sinervo drew this sketch of lizards in milk bottles to symbolize my ‘migration’ from lizards to Drosophila.
My first study with Drosophila was with Linda Patridge and Kevin Fowler. We showed that the thermal sensitivity of D. melanogaster evolves quickly when flies evolve by ‘laboratory natural selection’ at different temperatures. Cavicchi et al., 1995 reported results thermal divergence for other D. melanogaster evolving at three tempratures. Interestingly, only the high-temperature line had lost acclimation capacity, suggesting that selection for tolerance of high temperature causes a loss of acclimation to high temperature. Similar resuts have since been seen in other organisms.
Subsequently, several of us developed a simple way to measure “knock-down temperatures” (a non-lethal estimate of heat tolerance) of hundreds of flies. We adapted an apparatus designed by Ken Weber to select on ethanol resistance. Then using this apparatus, Gilchrist and Huey showed that knock-down temperature evolve rapidly in response to artificial selection and that knock-down temperature seemed to be governed by a major gene.
My decision to pursue laboratory studies of evolution was initially inspired by pioneering work of Michael Rose on aging and life history. Although I still appreciate the power and relevance of laboratory evolution, Huey and Rosenzweig(2009) presented a cautionary analysis of laboratory evolution studies.
In addition, we worked extensively on acclimation and cross-generational effects of temperature with Drosophila (Crill et al. 1996 and Huey et al. 1995). Huey et al. 1999 developed a strong inference approach (experimental design, statistical methodology) for evaluating the adaptive significance (or lack thereof) of acclimation.
The above studies were experimental and done in the laboratory. But given my background as a field biologist, I wanted to evaluate the rapidity of evolution in nature not merely in the lab. I found a remarkable series of papers by Antonio Prevosti and colleagues at the Universitat de Barcelona. They had shown that a fly (Drosophila subobscura), which was widely distributed in the Old World, had been introduced into both North and South America, had spread rapidly up and down the coasts of these continents, and was quickly evolving latitudinal clines in chromosomal inversion frequencies that were in the same direction as those in the Old World! This seemed like a great system to assay rapid evolution in nature of morphology and physiology.
I was lucky to join forces with them, and we published a series of studies on the theme of rapid evolution in nature. For example, Huey et al. 2000 showed that these flies had rapidly evolved body size clines – in the same direction with latitude as in the native Old World populations – in both North and in South America. Other studies monitored continuing clinal shifts in chromosomal inversions Balanya et al., 2003 and even within-locality shifts in inversion frequencies in response to global warming Balanya et al. 2006. Working with the team from Barcelona was a privledge and pleasure.
*Allometric engineering
Allometric studies have a long history in evolution and comparative biology. However, all involve inter-specific comparisons. Barry Sinervo (my former grad student and postdoc) pioneered an experimental way to manipulate body size of lizards, and we called this “allometric engineering.” Barry had experimental ‘hands of gold’ (actually he had ‘hands of bold’) and could miniaturize lizard eggs by sucking yolk from freshly laid eggs, and he could also make large eggs by ablating a few developing follicles in females. Sinervo and Huey, 1990 used yolk-sucking to show that inter-populational differences in speed of hatchling lizards was merely an effect of differences in egg size – a maternal effect. In contrast, differences in stamina of hatchlings largely persisted despite size reduction. Sinervo et al. 1992 later used allometric engineering to evaluate the effect of egg size on offspring survival in nature.
*Paleobiology
I’ve had an almost life-long interest is in paleobiology. When I was about 5 or 6 years old, my family visited the La Brea Tar Pits near Los Angeles, CA. I was fascinated by the fossils and announced that I wanted to become a paleontologist when I grew up. My Mother cautioned against this on the grounds that “there’s no money in it.” So I became a biologist.
Still, I remain fascinated by paleobiology and have done some work in this field. I worked with C. Richard Tracy and J. Scott Turner on a biophysical analysis of heat transfer in sailed pelycosaurs (Dimetrodon).
Huey and Ward 2005 looked that the physiological and biogeographic consequences of changes in oxygen levels over the Phanerozoic. This modeling study was inspired by Robert Berner’s pioneering work on variation in oxygen levels over geological time. Our simulations suggested that Pangea was not a super-highway because even foothills would have been physiological barriers to dispersal, given very low oxygen at that time. This was (for me) one of my most original papers.
We also used a similar approach to evaluate how high hypothetical “paleomountaineers” would have done on Mt. Everest over the Phanerozoic (see below).
For decades mountaineers considering an attempt on the great peaks of the world had no access to quantitative information on the risks or odds of success of climbing on various peaks, routes, or seasons. Much of what was reported was statistically wrong. But for peaks in Nepal (including Mt. Everest), those risks and odds can now be calculated – thanks to Elizabeth Hawley, who was a Reuters Correspondent in Kathmandu (she died in 2018 at age 94). Richard Salisbury compiled her archival data into The Himalayan Database, which is a freely accessible and updated twice yearly. If you like data and mountaineering, this is The Resource.
With several colleagues, I’ve pioneered studies that use The Himalayan Database to compute the odds of success (summiting, or summiting and surviving) and of death. For example, Huey and Eguskitza 2000 found that climbers who used supplemental oxygen had much lower death rates when descending from the summit of Mt. Everest or from K2 than did climbers who did not use supplemental oxygen, even though only the best and most experienced climbers can summit these peaks without supplemental oxygen.
Huey et al. 2008 examined how age and gender affected rates of success and dates on Mt. Everest. Huey et al. 2021 recently updated those analyses. The bottom lines of both papers are that men and women have very similar rates of success and of death, but that older climber have markedly low rates of summiting as well as markedly elevated death rates. Ray concedes that he missed his window of opportunity for Mt. Everest!
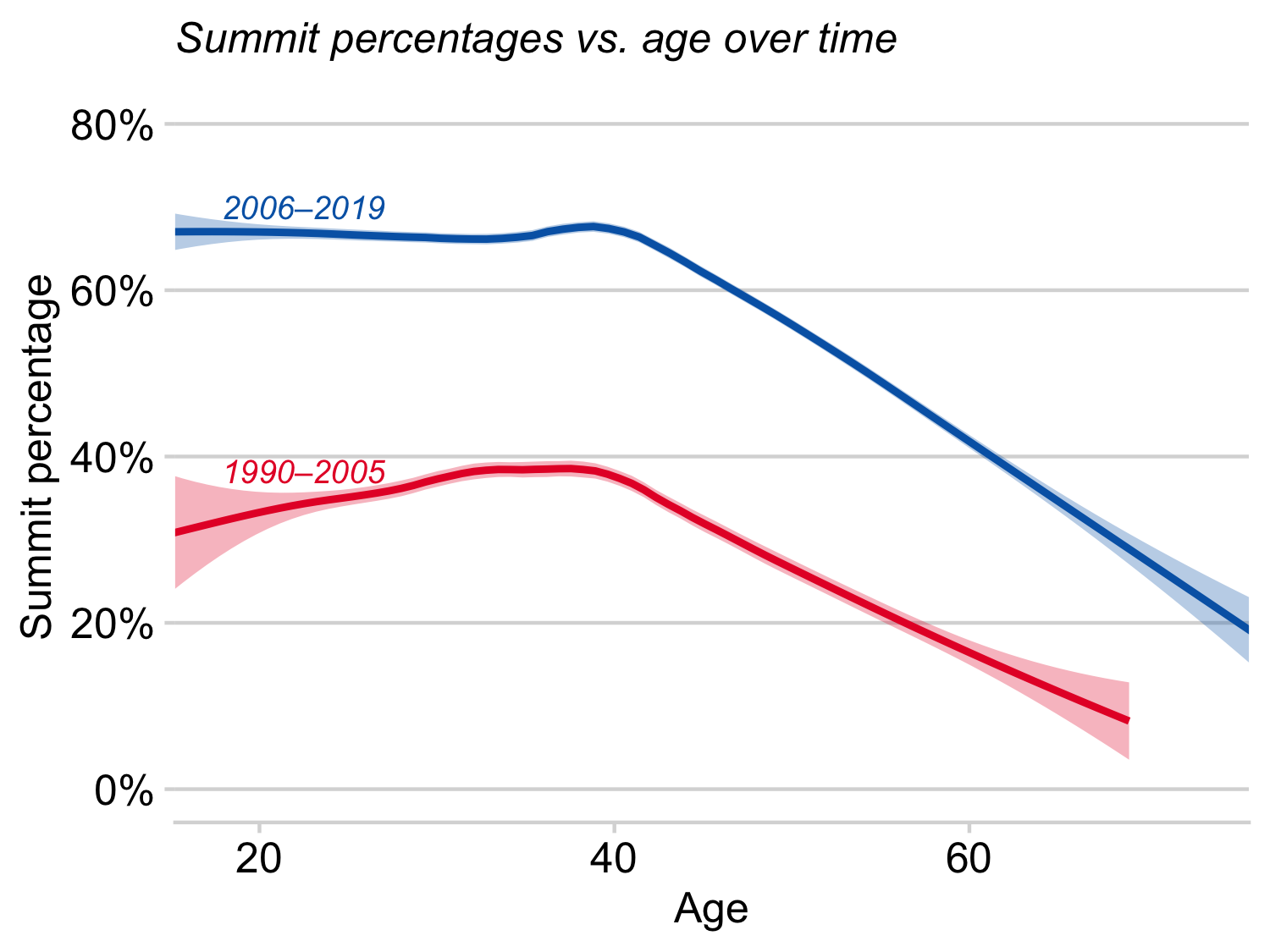
Rates of summiting versus age of climber in two successive time periods on Mt. Everest. In both periods, climbers older than about 40 had low success rates.
Death rates of climbers descending from the summit of Mt. Everest in two time periods. Few old climbers summit, and those that do have much higher death rates during descent than do young climbers.
I’ve published other studies on Himalayan mountaineering. These include a fun economic analysis of permit fees as a function of mountain height, seasonal variation in rates of success and death on Mt Everest, and on maximum altitude that ‘paleo-mountaineers’ could reach over the last 560 million years. This simulation used data from Robert Berner on oxygen levels over geological time. In the oxygen-rich Permian, we estimated that mountaineers could have climbed 1/3 higher than Mt. Everest. But in the oxygen-poor Triassic, they could not have reached an altitude equivalent to contemporary base camp on Mt. Everest. This is one of my favorite papers (and was my second paper in JAMA).
The predicted maximal altitude hypothetical “paleo-mountaineers” could have climbed (without supplemental oxygen) over the Phanerozoic. The shaded areas indicate periods of time when oxygen levels were sufficiently high to enable paleo-mountaineers to summit Mt. Everest.
As a kid (and now as an adult) I’ve treasured spending time on trails and peaks – but I was never a real climber. In any case, I have thoroughly enjoyed doing these mountaineering projects. Our findings are relevant to climbers and their friends and families.
On a personal level, these studies have given me an opportunity to meet and interact with Himalayan mountaineers – they are special breed. Their passion and commitment is infectious.
I don’t have children and so have never had to “brood” about naming a baby. Nonetheless, I became interested in whether climate and geography might influence the names parents give to their babies. Huey and Miles, 2022 focused on “spring” month names (April, May, June), which are associated with seasonality. We found that April was relatively common in southern states (USA), where spring weather comes early in the season, but that June was relatively common in northern states, where spring weather comes much later. When we focused on season names, we found that Autumn was most common in states with vivid autumn foliage. Overall, our studies show that climate can have surprising effects on human behavior. Elizabeth Pennisi wrote a Perspective in Science about this work.
NON-RESEARCH CONTRIBUTIONS
When Steve Stearns and I were Miller Postdocs in autumn 1976, we gave back-to-back talks on how to be a graduate student. Our contrasting (but complementary!) advice has proven of interest to graduate students for more than 1/2 century. In 2011, I took a fresh look at career advice – these reflections coming after a long career in academia.
I have worked to establish awards for young scientists, as such awards can give a significant boost to their careers. I am most proud of proposing and helping establish the George A. Bartholomew Award for Young Investigators (originally at American Society of Zoologists, currently Society for Integrative and Comparative Biology – SCIB). This annual award honors young investigators in comparative physiology, comparative biochemistry, integrative biology, or relate fields. Awardees give a plenary lecture at the annual SICB meetings. The award is named after Professor George A. Bartholomew, a founding leader in physiological ecology, integrative biology, and education. Bart had a special talent for synthesizing the philosophical basis of integrative science into a few sentences, and Al Bennett and I had great fun compiling some of Bart’s insights and prose.
Here at the University of Washington, I established the Ingrith Deyrup-Olsen Teaching Award (Deparment of Biology). This award honors the best Teaching Assistant (graduate student) in the Department and is named after Professor Deyrup-Olsen, a pioneer in STEM teaching, science education, and diversity.
The Division of Ecology and Evolution (SICB) established a best paper and best poster award for graduate students and named it after me (Raymond B. Huey Award). I myself had received a best-presentation award (Stoye Award, ASIH) when I was grad student, and thus I appreciate how important such award can be in bolstering the career of a young scientist. I thank my colleagues (especially Mike Angilletta and Art Woods) in the Division of Ecology & Evolution at SICB for the special honor.
My service contributions can be found in my CV.